Crystallization of polymers from solution occurs usually during the electrospinning process. At the moment we will discuss some very general aspects of solution crystallization. Crystallization is in general an example of a separation process in which mass is transferred from a liquid phase (solution or melt) to a solid crystal. The thermodynamic driving force for crystal nucleation and growth is the concentration excess (supersaturation) of the solution above the equilibrium (saturation). Once crystallization is occurred, equilibrium is set between the crystals and the residual mother liquid, the balance being determined by the solubility (concentration) and the temperature. The resistances to growth are related to mass transfer within the solution and the energy needed at the crystal surface for incoming molecules to orient themselves to the crystal lattice.
Solution crystallization is a complex process being governed by various parameters. The first one is a solubility which is a function of temperature and related saturation. For most materials solubility increases with temperature (Fig. 1).
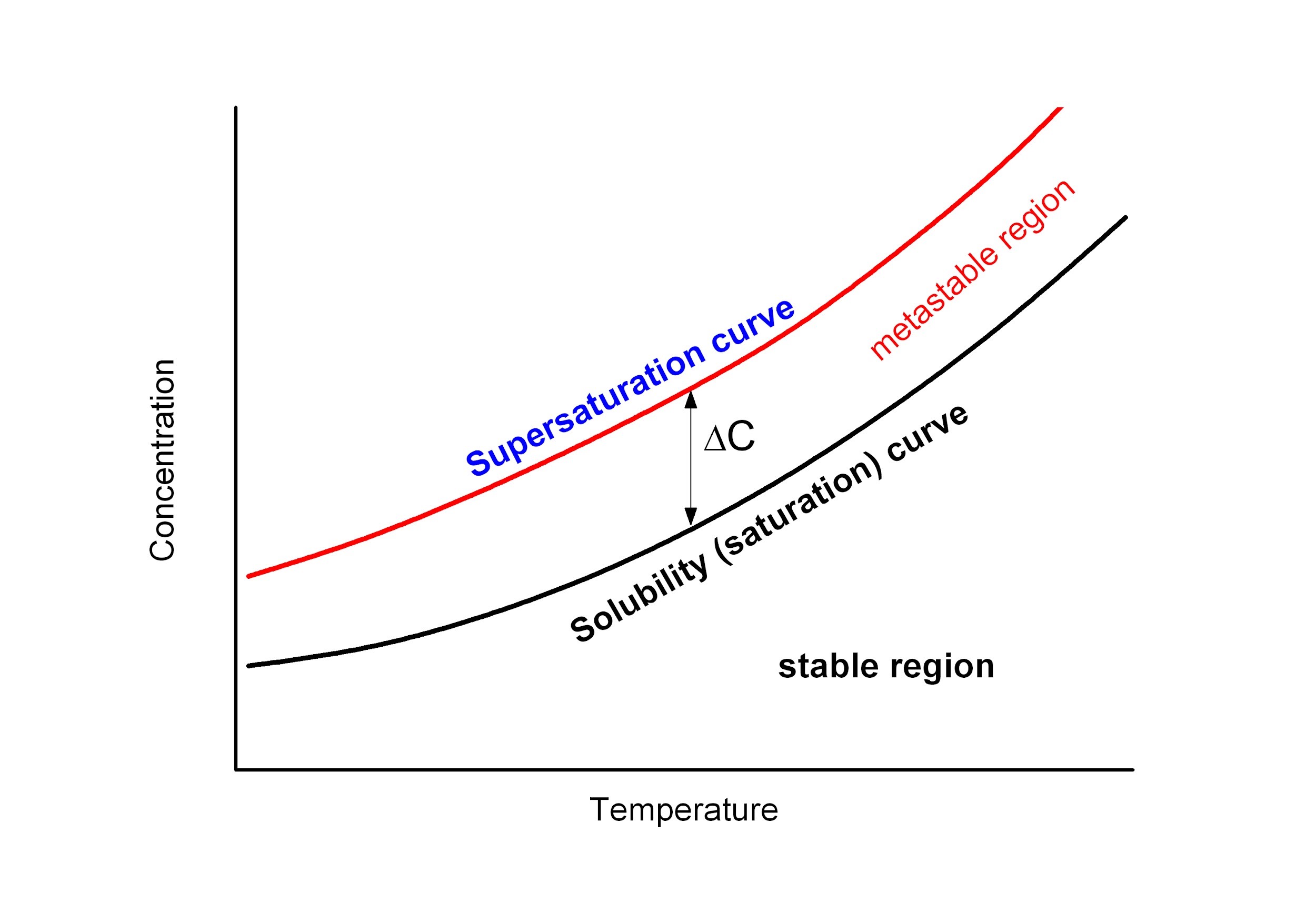
Considering solution crystallization at constant temperature, it starts after solvent evaporation being sufficient to cross the saturation curve. Immediately after crossing saturation curve, there is metastable region in which spontaneous nucleation is immposible because of the role of interfacial energy being in opposition to the driving volume free energy. In the metastable region, there is an equilibrium between the processes of crystal formation and dispersion. Above the next saturation line, called supersaturation, crystals can form spontaneously (homogeneously. During crystallization, the crystals are grown from solutions with concentrations higher than the saturation level in the solubility curves. Once nuclei are formed the crystals will continue to grow so long as supersaturation exists. There are several factors controlling the rates of crystal nucleation (primary nucleation) and growth (secondary nucleation). The most important is the degree of supersaturation, viscosity, interfacial tension between the solute and the solvent, boiling temperature of a solvent as well as strength of a solvent which affects position of the saturation line.
Supersaturation is critical because it is the driving force for crystal nucleation – either spontaneously (primary nucleation) or in the presence of existing crystals (secondary nucleation - growth). Crystal growth is the increase in size of crystals as solute is deposited from solution. These often competing mechanisms ultimately determine the final crystal size distribution. The relationship between supersaturation, ΔC, and rate of nucleation, N, and growth, G, can be defined by simplified equations:
where kg is growth constant, g - growth order, kn - nucleation constant, n - nucleation order. For organic crystallization systems, the value of the growth order is typically between 1 and 2 and the value of the nucleation order is typically between 5 and 10, leading to the scheme drawn in Fig. 2.
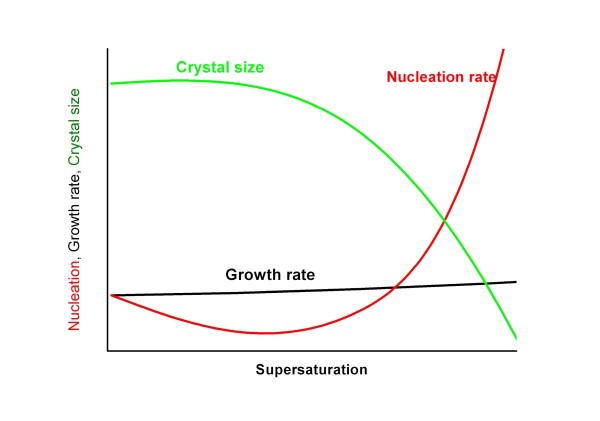
At low supersaturation, crystals can grow faster than they nucleate resulting in a larger crystal size distribution. However, at higher supersaturation, crystal nucleation dominates crystal growth, ultimately resulting in smaller crystals. This is a problem of relatively low total thermodynamic driving force (high positive input of surface free energy being in opposition to the negative volume free energy) at low supersaturation which can be enhanced by nucleation on earlier formed crystals (growth). If supersaturation is high, there may be further effective nucleation and the growth will not be so great. In practice, slow evaporation rate maintaining a low level of supersaturation produces large crystals and fast evaporation results in formation rather small/defective crystals.
Additional parameter involved in thermodynamics of solvent crystallization is interfacial tension between the solute and the solvent. In the case of solvents providing high interfacial tension between the solute and the solvent, there will be higher surface barrier against crystallization. It will lead to retardation of crystallization, particularly evident for the stage of primary nucleation.
Viscosity of solution is crucial for molecular transport which is needed for crystal growth. The rate of crystal growth is controlled by the diffusion of the solute through the solvent to the surface of the crystal and by the rate of the reaction at the crystal face when the solute molecules rearrange themselves into the crystal lattice. These rates of crystal growth can be represented by the equations
where dw is the increase in weight of crystals in time dt, A is the surface area of the crystals, c is the solute concentration of the bulk solution, ci is the solute local concentration at the crystal/solution interface, cs is the concentration of the saturated solution, Kd is the mass transfer coefficient to the interface and Ks is the rate constant for the surface reaction. The equations are usually combined into more simple final form:
where
It is evident that the growth is faster for higher values of K, which is inversely proportional to the viscosity.
Additionally discussing solution viscosity, one should be aware that it reflects the strength of solvent for particular polymer. The higher is the viscosity for given polymer the better is the solvent. This fact can be seen from Mark-Houwink equation, providing relation between solution intrinsic visocity [h] and polymer molecular weight, M:
in which the exponent “a” depends strongly on a polymer configuration. In a “poor” solvent, the segments of a polymer molecule attract each other in solution more strongly than they attract the surrounding solvent molecules. The polymer molecule assumes then a tighter configuration, and the solution has a lower intrinsic viscosity resulting in Mark-Houwink “a” constant close to 0.5. For better solvents, the molecules tend to adopt more extended configuration, resulting in higher “a” constant values (higher viscosity).
Furthermore, the better is solvent the higher is saturation concentration (higher positions of saturation lines on Fig. 1). This fact can be important for the final crystal structure because of shorter time accessible for crystallization in the case of better solvents. Similar effects can be expected for solvents with low boiling points.